The dynamics of blood vessels and vascular prostheses
A paradigmatic shift is needed to tackle the study of cardiovascular mechanics from a dynamic perspective rather than a static one. This research operates at the interface between fluid-structure interaction and cardiovascular solid mechanics with the objective to expand the knowledge about physiology and pathophysiology of the vasculature, and to improve the requisite diagnostic and therapeutic capabilities.
- It is well known that vascular diseases are associated with changes in the mechanical properties of the arterial wall; however, some disorders such as aortic dissection and rupture have no biomechanical explanation yet. To identify critical regions where local aortic wall remodeling and blood flow alteration may happen, the Tubaldi Lab develops advanced fluid-structure interaction models and constantly improve them by comparing against ex-vivo experiments and in-vivo data.
- In vascular surgery, the design of synthetic vascular prostheses with the same dynamical physical properties of human arteries still represents an open question. Currently used Dacron grafts to treat patients with aortic dissection and aneurysms are known to alter hemodynamic parameters at the implant site, in both the proximal and distal regions, and to increase the heart workload. This research aims to design the next generation prosthesis able (i) to mimic the dynamic behavior of native vessels, (ii) to resist dilation, and (iii) to permit arterial pulsatile flow.
Nonlinear mechanical metamaterials
Metamaterials are materials whose properties emerge from structure rather than composition. In the Tubaldi Lab, we do not modify any material at the molecular level, but we assemble reasonably simple building blocks in a unique fashion to obtain some specific and unusual behaviors. Our current research project on snapping of shallow arches is opening avenues on the fascinating world of transition waves in multistable lattices. We are harnessing chains of bistable shallow arches to transmit mechanical signals without distortion at a potentially infinite distance.
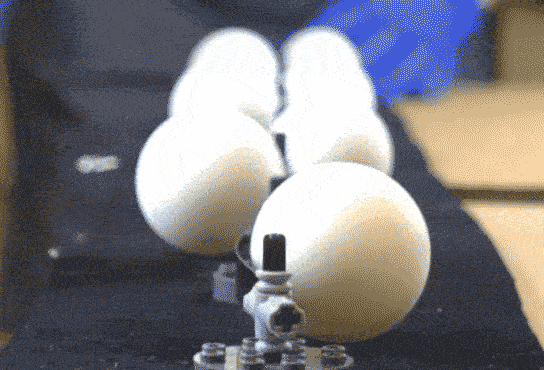
Soft bioinspired robotics
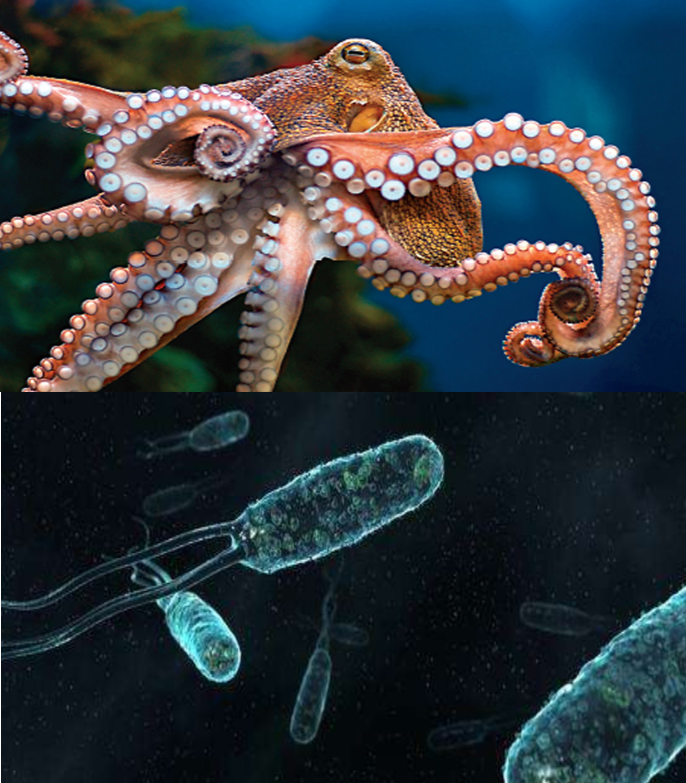
With their soft biological tissues, living creatures can perform complex tasks which are impossible to mimic with artificial mechanical rigid systems. An octopus can squeeze through a hole as small as its eyes while bacteria can propel themselves through their flexible flagella. Creative engineering designs are often inspired by nature, which over millions of years of evolution has optimized structures to best fit their surroundings. Elastomeric soft robots can replicate convoluted biomimetic motion using a simple fluidic pressure actuation. Yet, accurately designing and controlling soft actuators remains an open issue due to material nonlinearities. The Tubaldi Lab investigates the still unknown nonlinear dynamics of bioinspired soft robots with fast fluidic actuation or with external fluid field excitation.